From The University of California-Berzerkeley
July 26, 2022
Robert Sanders
rlsanders@berkeley.edu
A spinning neutron star periodically swings its radio (green) and gamma-ray (magenta) beams past Earth in this artist’s concept of a black widow pulsar. The neutron star/pulsar heats the facing side of its stellar partner (right) to temperatures twice as hot as the sun’s surface and slowly evaporates it. (Image credit: NASA’s Goddard Space Flight Center)
A dense, collapsed star spinning 707 times per second — making it one of the fastest spinning neutron stars in the Milky Way galaxy — has shredded and consumed nearly the entire mass of its stellar companion and, in the process, grown into the heaviest neutron star observed to date.
Weighing this record-setting neutron star, which tops the charts at 2.35 times the mass of the sun, helps astronomers understand the weird quantum state of matter inside these dense objects, which — if they get much heavier than that — collapse entirely and disappear as a black hole.
“We know roughly how matter behaves at nuclear densities, like in the nucleus of a uranium atom,” said Alex Filippenko, Distinguished Professor of Astronomy at the University of California-Berzerkeley. “A neutron star is like one giant nucleus, but when you have one-and-a-half solar masses of this stuff, which is about 500,000 Earth masses of nuclei all clinging together, it’s not at all clear how they will behave.”
Roger W. Romani, professor of astrophysics at Stanford University, noted that neutron stars are so dense — 1 cubic inch weighs over 10 billion tons — that their cores are the most dense matter in the universe short of black holes, which because they are hidden behind their event horizon are impossible to study. The neutron star, a pulsar designated PSR J0952-0607 is thus the densest object within sight of Earth.
The measurement of the neutron star’s mass was possible thanks to the extreme sensitivity of the 10-meter Keck I telescope on Mauna Kea in Hawai’i, which was just able to record a spectrum of visible light from the hotly glowing companion star, now reduced to the size of a large gaseous planet. The stars are about 3,000 light years from Earth in the direction of the constellation Sextans.
Astronomers measured the velocity of a faint star (green circle) that has been stripped of nearly its entire mass by an invisible companion, a neutron star and millisecond pulsar that they determined to be the most massive yet found and perhaps the upper limit for neutron stars. Image credit: Roger W. Romani, Alex Filippenko/ W. M. Keck Observatory.
Discovered in 2017, PSR J0952-0607 [The Astrophysical Journal Letters (below)] is referred to as a “black widow” pulsar — an analogy to the tendency of female black widow spiders to consume the much smaller male after mating. Filippenko and Romani have been studying black widow systems for more than a decade, hoping to establish the upper limit on how large neutron stars/pulsars can grow.
“By combining this measurement with those of several other black widows, we show that neutron stars must reach at least this mass, 2.35 plus or minus 0.17 solar masses,” said Romani, who is a professor of physics in Stanford’s School of Humanities and Sciences and member of the Kavli Institute for Particle Astrophysics and Cosmology. “In turn, this provides some of the strongest constraints on the property of matter at several times the density seen in atomic nuclei. Indeed, many otherwise popular models of dense-matter physics are excluded by this result.”
If 2.35 solar masses is close to the upper limit of neutron stars, the researchers say, then the interior is likely to be a soup of neutrons as well as up and down quarks — the constituents of normal protons and neutrons — but not exotic matter, such as “strange” quarks or kaons, which are particles that contain a strange quark.
“A high maximum mass for neutron stars suggests that it is a mixture of nuclei and their dissolved up and down quarks all the way to the core,” Romani said. “This excludes many proposed states of matter, especially those with exotic interior composition.”
Romani, Filippenko and Stanford graduate student Dinesh Kandel are co-authors of a paper describing the team’s results that has been accepted for publication by The Astrophysical Journal Letters [below].
How large can they grow?
Astronomers generally agree that when a star with a core larger than about 1.4 solar masses collapses at the end of its life, it forms a dense, compact object with an interior under such high pressure that all atoms are smashed together to form a sea of neutrons and their subnuclear constituents, quarks. These neutron stars are born spinning, and though too dim to be seen in visible light, reveal themselves as pulsars, emitting beams of light — radio waves, X-rays or even gamma rays — that flash Earth as they spin, much like the rotating beam of a lighthouse.
A Black Widow Pulsar Consumes its Mate. NASA Goddard.
This 2014 NASA video explains black widow pulsars and how astronomers discovered one called PSR J1311−3430, the first of its kind discovered solely through gamma-ray observations. (Video courtesy of NASA’s Goddard Space Flight Center)
“Ordinary” pulsars spin and flash about once per second, on average, a speed that can easily be explained given the normal rotation of a star before it collapses. But some pulsars repeat hundreds or up to 1,000 times per second, which is hard to explain unless matter has fallen onto the neutron star and spun it up. But for some millisecond pulsars, no companion is visible.
One possible explanation for isolated millisecond pulsars is that each did once have a companion, but it stripped it down to nothing.
“The evolutionary pathway is absolutely fascinating. Double exclamation point,” Filippenko said. “As the companion star evolves and starts becoming a red giant, material spills over to the neutron star, and that spins up the neutron star. By spinning up, it now becomes incredibly energized, and a wind of particles starts coming out from the neutron star. That wind then hits the donor star and starts stripping material off, and over time, the donor star’s mass decreases to that of a planet, and if even more time passes, it disappears altogether. So, that’s how lone millisecond pulsars could be formed. They weren’t all alone to begin with — they had to be in a binary pair — but they gradually evaporated away their companions, and now they’re solitary.”
The pulsar PSR J0952-0607 and its faint companion star support this origin story for millisecond pulsars.
“These planet-like objects are the dregs of normal stars which have contributed mass and angular momentum, spinning up their pulsar mates to millisecond periods and increasing their mass in the process,” Romani said.
“In a case of cosmic ingratitude, the black widow pulsar, which has devoured a large part of its mate, now heats and evaporates the companion down to planetary masses and perhaps complete annihilation,” said Filippenko.
Spider pulsars include redbacks and tidarrens
Finding black widow pulsars in which the companion is small, but not too small to detect, is one of few ways to weigh neutron stars. In the case of this binary system, the companion star — now only 20 times the mass of Jupiter — is distorted by the mass of the neutron star and tidally locked, similar to the way our moon is locked in orbit so that we see only one side. The neutron star-facing side is heated to temperatures of about 6,200 Kelvin, or 10,700 degrees Fahrenheit, a bit hotter than our sun, and just bright enough to see with a large telescope.
Filippenko and Romani turned the Keck I telescope on PSR J0952-0607 on six occasions over the last four years, each time observing with the Low Resolution Imaging Spectrometer in 15-minute chunks to catch the faint companion at specific points in its 6.4-hour orbit of the pulsar.

By comparing the spectra to that of similar sun-like stars, they were able to measure the orbital velocity of the companion star and calculate the mass of the neutron star.
Filippenko and Romani have examined about a dozen black widow systems so far, though only six had companion stars bright enough to let them calculate a mass. All involved neutron stars less massive than the pulsar PSR J0952-060. They’re hoping to study more black widow pulsars, as well as their cousins: redbacks, named for the Australian equivalent of black widow pulsars, which have companions closer to one-tenth the mass of the sun; and what Romani dubbed tidarrens — where the companion is around one-hundredth of a solar mass — after a relative of the black widow spider. The male of this species, Tidarren sisyphoides, is about 1% of the female’s size.
“We can keep looking for black widows and similar neutron stars that skate even closer to the black hole brink. But if we don’t find any, it tightens the argument that 2.3 solar masses is the true limit, beyond which they become black holes,” Filippenko said.
“This is right at the limit of what the Keck telescope can do, so barring fantastic observing conditions, tightening the measurement of PSR J0952-0607 likely awaits the 30-meter telescope era,” added Romani.
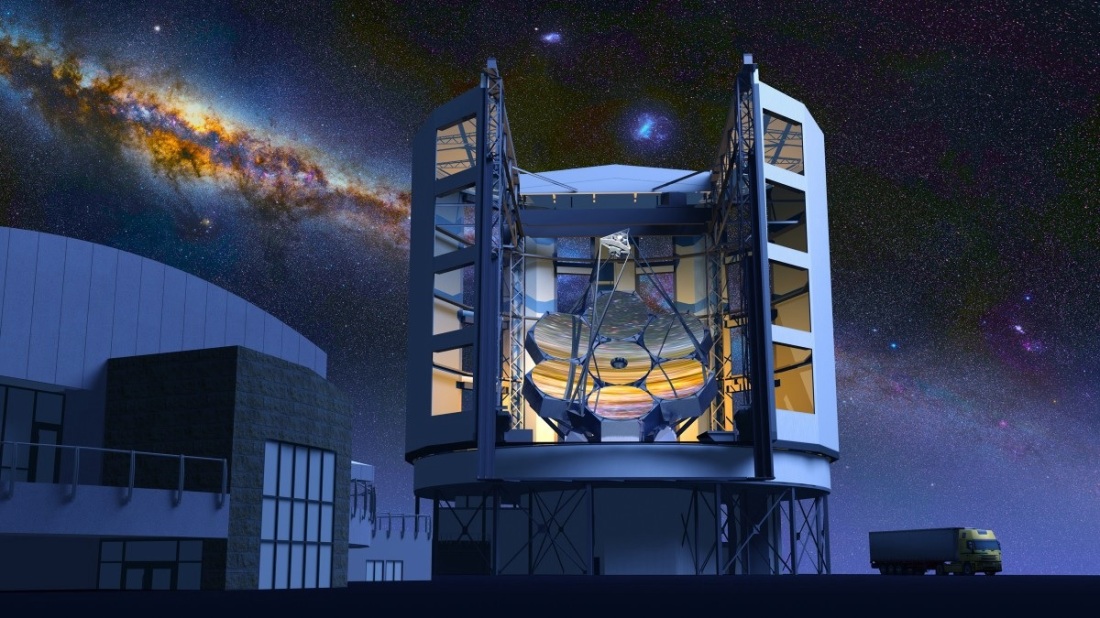
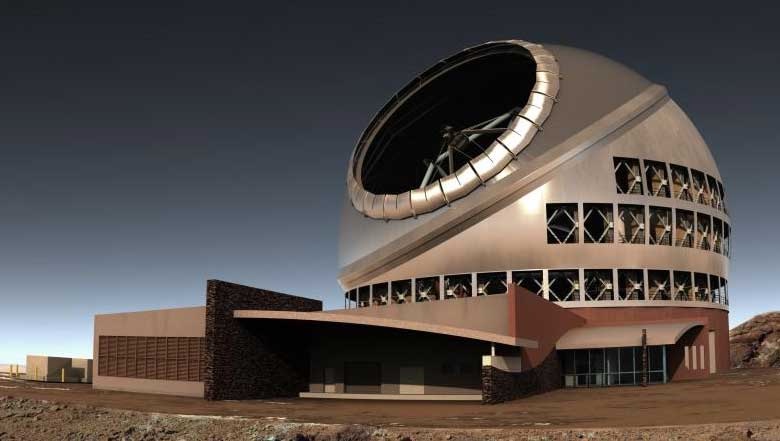

Other co-authors of the ApJL paper are UC Berzerkeley researchers Thomas Brink and WeiKang Zheng. The work was supported by the National Aeronautics and Space Administration (80NSSC17K0024, 80NSSC17K0502), the Christopher R. Redlich Fund, the TABASGO Foundation, and UC Berzerkeley’s Miller Institute for Basic Research in Science.
Science papers:
The Astrophysical Journal Letters 2017
The Astrophysical Journal Letters 2022
_______________________________________________
Women in STEM – Dame Susan Jocelyn Bell Burnell Discovered pulsars



Biography
British astrophysicist, scholar and trailblazer Jocelyn Bell Burnell discovered the space-based phenomena known as pulsars, going on to establish herself as an esteemed leader in her field. Who Is Jocelyn Bell Burnell?
Jocelyn Bell Burnell is a British astrophysicist and astronomer. As a research assistant, she helped build a large radio telescope and discovered pulsars, providing the first direct evidence for the existence of rapidly spinning neutron stars. In addition to her affiliation with Open University, she has served as dean of science at the University of Bath and president of the Royal Astronomical Society. Bell Burnell has also earned countless awards and honors during her distinguished academic career.
Early Life
Jocelyn Bell Burnell was born Susan Jocelyn Bell on July 15, 1943, in Belfast, Northern Ireland. Her parents were educated Quakers who encouraged their daughter’s early interest in science with books and trips to a nearby observatory. Despite her appetite for learning, however, Bell Burnell had difficulty in grade school and failed an exam intended to measure her readiness for higher education.
Undeterred, her parents sent her to England to study at a Quaker boarding school, where she quickly distinguished herself in her science classes. Having proven her aptitude for higher learning, Bell Burnell attended the University of Glasgow, where she earned a bachelor’s degree in physics in 1965.
Little Green Men
In 1965, Bell Burnell began her graduate studies in radio astronomy at Cambridge University. One of several research assistants and students working under astronomers Anthony Hewish, her thesis advisor, and Martin Ryle, over the next two years she helped construct a massive radio telescope designed to monitor quasars. By 1967, it was operational and Bell Burnell was tasked with analyzing the data it produced. After spending endless hours pouring over the charts, she noticed some anomalies that did not fit with the patterns produced by quasars and called them to Hewish’s attention.
Over the ensuing months, the team systematically eliminated all possible sources of the radio pulses—which they affectionately labeled Little Green Men, in reference to their potentially artificial origins—until they were able to deduce that they were made by neutron stars, fast-spinning collapsed stars too small to form black holes.
Pulsars and Nobel Prize Controversy
Their findings were published in the February 1968 issue of Nature and caused an immediate sensation. Intrigued as much by the novelty of a woman scientist as by the astronomical significance of the team’s discovery, which was labeled pulsars—for pulsating radio stars—the press picked up the story and showered Bell Burnell with attention. That same year, she earned her Ph.D. in radio astronomy from Cambridge University.
However, in 1974, only Hewish and Ryle received the Nobel Prize for Physics for their work. Many in the scientific community raised their objections, believing that Bell Burnell had been unfairly snubbed. However, Bell Burnell humbly rejected the notion, feeling that the prize had been properly awarded given her status as a graduate student, though she has also acknowledged that gender discrimination may have been a contributing factor.
Life on the Electromagnetic Spectrum
Nobel Prize or not, Bell Burnell’s depth of knowledge regarding radio astronomy and the electromagnetic spectrum has earned her a lifetime of respect in the scientific community and an esteemed career in academia. After receiving her doctorate from Cambridge, she taught and studied gamma ray astronomy at the University of Southampton. Bell Burnell then spent eight years as a professor at University College London, where she focused on x-ray astronomy.
During this same time, she began her affiliation with Open University, where she would later work as a professor of physics while studying neurons and binary stars, and also conducted research in infrared astronomy at the Royal Observatory, Edinburgh. She was the Dean of Science at the University of Bath from 2001 to 2004, and has been a visiting professor at such esteemed institutions as Princeton University and Oxford University.
Array of Honors and Achievements
In recognition of her achievements, Bell Burnell has received countless awards and honors, including Commander and Dame of the Order of the British Empire in 1999 and 2007, respectively; an Oppenheimer prize in 1978; and the 1989 Herschel Medal from the Royal Astronomical Society, for which she would serve as president from 2002 to 2004. She was president of the Institute of Physics from 2008 to 2010, and has served as president of the Royal Society of Edinburgh since 2014. Bell Burnell also has honorary degrees from an array of universities too numerous to mention.
Personal Life
In 1968, Jocelyn married Martin Burnell, from whom she took her surname, with the two eventually divorcing in 1993. The two have a son, Gavin, who has also become a physicist.
A documentary on Bell Burnell’s life, Northern Star, aired on the BBC in 2007.
Dame Susan Jocelyn Bell Purnell at Perimeter Institute Oct 26, 2018.
_______________________________________________
See the full article here .
five-ways-keep-your-child-safe-school-shootings
Please help promote STEM in your local schools.
The University of California-Berzerkeley is a public land-grant research university in Berkeley, California. Established in 1868 as the state’s first land-grant university, it was the first campus of the University of California system and a founding member of the Association of American Universities . Its 14 colleges and schools offer over 350 degree programs and enroll some 31,000 undergraduate and 12,000 graduate students. Berkeley is ranked among the world’s top universities by major educational publications.
Berzerkeley hosts many leading research institutes, including the Mathematical Sciences Research Institute and the Space Sciences Laboratory. It founded and maintains close relationships with three national laboratories at DOE’s Lawrence Berkeley National Laboratory, DOE’s Lawrence Livermore National Laboratory and DOE’s Los Alamos National Lab, and has played a prominent role in many scientific advances, from the Manhattan Project and the discovery of 16 chemical elements to breakthroughs in computer science and genomics. Berzerkeley is also known for student activism and the Free Speech Movement of the 1960s.
Berzerkeley alumni and faculty count among their ranks 110 Nobel laureates (34 alumni), 25 Turing Award winners (11 alumni), 14 Fields Medalists, 28 Wolf Prize winners, 103 MacArthur “Genius Grant” recipients, 30 Pulitzer Prize winners, and 19 Academy Award winners. The university has produced seven heads of state or government; five chief justices, including Chief Justice of the United States Earl Warren; 21 cabinet-level officials; 11 governors; and 25 living billionaires. It is also a leading producer of Fulbright Scholars, MacArthur Fellows, and Marshall Scholars. Berzerkeley alumni, widely recognized for their entrepreneurship, have founded many notable companies.
Berzerkeley’s athletic teams compete in Division I of the NCAA, primarily in the Pac-12 Conference, and are collectively known as the California Golden Bears. The university’s teams have won 107 national championships, and its students and alumni have won 207 Olympic medals.
Made possible by President Lincoln’s signing of the Morrill Act in 1862, the University of California was founded in 1868 as the state’s first land-grant university by inheriting certain assets and objectives of the private College of California and the public Agricultural, Mining, and Mechanical Arts College. Although this process is often incorrectly mistaken for a merger, the Organic Act created a “completely new institution” and did not actually merge the two precursor entities into the new university. The Organic Act states that the “University shall have for its design, to provide instruction and thorough and complete education in all departments of science, literature and art, industrial and professional pursuits, and general education, and also special courses of instruction in preparation for the professions”.
Ten faculty members and 40 students made up the fledgling university when it opened in Oakland in 1869. Frederick H. Billings, a trustee of the College of California, suggested that a new campus site north of Oakland be named in honor of Anglo-Irish philosopher George Berkeley. The university began admitting women the following year. In 1870, Henry Durant, founder of the College of California, became its first president. With the completion of North and South Halls in 1873, the university relocated to its Berkeley location with 167 male and 22 female students.
Beginning in 1891, Phoebe Apperson Hearst made several large gifts to Berkeley, funding a number of programs and new buildings and sponsoring, in 1898, an international competition in Antwerp, Belgium, where French architect Émile Bénard submitted the winning design for a campus master plan.
20th century
In 1905, the University Farm was established near Sacramento, ultimately becoming the University of California-Davis. In 1919, Los Angeles State Normal School became the southern branch of the University, which ultimately became the University of California-Los Angeles. By 1920s, the number of campus buildings had grown substantially and included twenty structures designed by architect John Galen Howard.
In 1917, one of the nation’s first ROTC programs was established at Berkeley and its School of Military Aeronautics began training pilots, including Gen. Jimmy Doolittle. Berkeley ROTC alumni include former Secretary of Defense Robert McNamara and Army Chief of Staff Frederick C. Weyand as well as 16 other generals. In 1926, future fleet admiral Chester W. Nimitz established the first Naval ROTC unit at Berkeley.
In the 1930s, Ernest Lawrence helped establish the Radiation Laboratory (now DOE’s Lawrence Berkeley National Laboratory (US)) and invented the cyclotron, which won him the Nobel physics prize in 1939. Using the cyclotron, Berkeley professors and Berkeley Lab researchers went on to discover 16 chemical elements—more than any other university in the world. In particular, during World War II and following Glenn Seaborg’s then-secret discovery of plutonium, Ernest Orlando Lawrence’s Radiation Laboratory began to contract with the U.S. Army to develop the atomic bomb. Physics professor J. Robert Oppenheimer was named scientific head of the Manhattan Project in 1942. Along with the Lawrence Berkeley National Laboratory, Berzerkeley founded and was then a partner in managing two other labs, The Doe’s Los Alamos National Laboratory (1943) and The DOE’s Lawrence Livermore National Laboratory (1952).
By 1942, the American Council on Education ranked Berzerkeley second only to Harvard University in the number of distinguished departments.
In 1952, the University of California reorganized itself into a system of semi-autonomous campuses, with each campus given its own chancellor, and Clark Kerr became Berkeley’s first Chancellor, while Sproul remained in place as the President of the University of California.
Berzerkeley gained a worldwide reputation for political activism in the 1960s. In 1964, the Free Speech Movement organized student resistance to the university’s restrictions on political activities on campus—most conspicuously, student activities related to the Civil Rights Movement. The arrest in Sproul Plaza of Jack Weinberg, a recent Berkeley alumnus and chair of Campus CORE, in October 1964, prompted a series of student-led acts of formal remonstrance and civil disobedience that ultimately gave rise to the Free Speech Movement, which movement would prevail and serve as precedent for student opposition to America’s involvement in the Vietnam War.
In 1982, the Mathematical Sciences Research Institute (MSRI) was established on campus with support from the National Science Foundation and at the request of three Berzerkeley mathematicians — Shiing-Shen Chern, Calvin Moore and Isadore M. Singer. The institute is now widely regarded as a leading center for collaborative mathematical research, drawing thousands of visiting researchers from around the world each year.
21st century
In the current century, Berzerkeley has become less politically active and more focused on entrepreneurship and fundraising, especially for STEM disciplines.
Modern Berzerkeley students are less politically radical, with a greater percentage of moderates and conservatives than in the 1960s and 70s. Democrats outnumber Republicans on the faculty by a ratio of 9:1. On the whole, Democrats outnumber Republicans on American university campuses by a ratio of 10:1.
In 2007, the Energy Biosciences Institute was established with funding from BP and Stanley Hall, a research facility and headquarters for the California Institute for Quantitative Biosciences, opened. The next few years saw the dedication of the Center for Biomedical and Health Sciences, funded by a lead gift from billionaire Li Ka-shing; the opening of Sutardja Dai Hall, home of the Center for Information Technology Research in the Interest of Society; and the unveiling of Blum Hall, housing the Blum Center for Developing Economies. Supported by a grant from alumnus James Simons, the Simons Institute for the Theory of Computing was established in 2012. In 2014, Berzerkeley and its sister campus, University of California-San Fransisco, established the Innovative Genomics Institute, and, in 2020, an anonymous donor pledged $252 million to help fund a new center for computing and data science.
Since 2000, Berzerkeley alumni and faculty have received 40 Nobel Prizes, behind only Harvard and Massachusetts Institute of Technology among US universities; five Turing Awards, behind only MIT and Stanford University; and five Fields Medals, second only to Princeton University. According to PitchBook, Berzerkeley ranks second, just behind Stanford University, in producing VC-backed entrepreneurs.