From JSTOR, nonprofit library for the intellectually curious
At
Nonprofit library for the intellectually curious
5.23.24
Adam Frank
A deep dive into the chaotic journey of star formation.
The stellar nursery called Sharpless 29 via The European Southern Observatory [La Observatorio Europeo Austral] [Observatoire européen austral][Europäische Südsternwarte](EU)(CL)
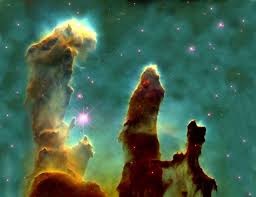
Like human births, the birth of stars is a messy affair. Beginning with vast interstellar clouds that collapse under their own titanic gravity, the story of how stars like the Sun are born involves great paroxysms of light and sound (yes, there is sound in space). But when the dust clears, (literally), what remains is a nuclear-powered stellar furnace that will burn brightly for billions of years surrounded by a family of planets using that same energy to do everything from powering climates to creating life. This star-formation story is one that astronomers have only recently assembled via hard-won observations made by the world’s most powerful telescopes. It’s a narrative that not only explains the star-strewn night sky but also puts Earth and its inhabitants into their proper cosmic context.
The Interstellar Medium
To understand the story of star formation, we need to set the cosmic stage. In the current cosmological epoch, much of the “normal matter” in the Universe (excluding the “dark” stuff) resides in galaxies like the Milky Way (though there’s still a lot of stuff residing in the vast regions between galaxies, too). Galaxies like ours comprise both stars and interstellar gas (also called the interstellar medium, or ISM). The Milky Way, for example, holds more than 100 billion stars and about 10 billion times the Sun’s worth of interstellar material. It’s the interstellar stuff that matters for making new stars and the ISM can take various forms.
Some interstellar gas is evenly distributed in sparse clouds of atomic hydrogen at “warm” temperatures of 8,000 kelvin. Another form of interstellar material is the ultra-hot, million-degree “coronal gas.” This material is composed of highly ionized atoms (i.e., atoms stripped of some electrons) left over from supernovas, which are apocalyptic explosions of stars much more massive than the Sun. However, neither the warm atomic nor hot coronal kinds of interstellar gas are directly involved in birthing a star. Both forms of ISM are, as we will see, too thinly spread out and at too high a temperature to allow the process of star formation to occur in their domains.
It’s interstellar gas in the form of molecular clouds that are the focus for star formation. That’s because these are the densest and coldest regions of the ISM. With temperatures just tens of degrees above zero, gas in these clouds can chemically combine to form things like carbon monoxide, ammonia, and molecular hydrogen (hence the “molecular” moniker). Once the molecules form, the density in the clouds is high enough to shield them from being split apart by the background stellar UV radiation that fills interstellar space.
So, why are low temperatures and high densities critical for star formation? The answer is the Universe’s “forever war” between gravity and pressure. Clouds are supported against their own weight—their own “self-gravity”—by thermal pressure. The pressure pushes outward, keeping a cloud “inflated,” just as a balloon is inflated by the thermal pressure of the trapped air pushing against its rubber skin. To convert interstellar material into a star, you need to gather enough mass together so that the sum of its own gravitational pull will overwhelm the cloud’s thermal pressure and trigger a gravitational collapse. The key physics point is that cold gas has less thermal pressure than hot gas. Equally important, a dense cloud has more self-gravity than an equally sized sparse cloud (more material per cubic meter equals more inward gravitational pull). Put it all together and the dense, cold molecular clouds are the only interstellar environment where gravitational collapse can occur. That’s why molecular clouds serve as stellar nurseries for the Milky Way.
Gravitational Collapse and the Birth of Protostars
Typical molecular clouds can hold over a million Suns’ worth of material. They can also stretch across hundreds of light-years. The sheer size of these clouds means molecular clouds don’t collapse as a single entity. If they did there would be stars weighing a million solar masses. Instead, the largest stars today are about 100 times the mass of the Sun. So, to study the formation of stars like the Sun, we need to focus on smaller regions of the larger molecular cloud. Molecular clouds are not static structures but are filled with turbulence (i.e. random swirling motions like eddies in a fast-moving river). The turbulence creates local conditions of high density—think of them as “cloudlets”—where self-gravity can overwhelm thermal pressure and trigger collapse. It’s at the moment when gravity begins to “win” that star formation really begins. Molecular gas and dust begin to fall inward toward the center of the cloudlet.
The gas and dust accelerate as they fall, eventually reaching speeds of more than 22,000 miles per hour. The infalling material also gets denser as it converges toward the cloudlet’s center. Initially, parcels of gas remain cold as they stream inward. Any heat gained from jostling and crowding as the gas plunges downward gets emitted as light. These photons can escape the shrinking cloudlet into space (and reach our telescopes). Eventually, however, the infalling material gets so dense it begins holding onto most of its own energy. This includes the heat generated as material crashes at hypersonic speeds onto the rapidly growing and dense central object, which now can rightfully be called a “protostar.” Powerful shock waves (a form of sound) form at the surface of the protostar as streams of gas slam onto its surface. While most of the energy generated from these shocks stays with the protostar, some energy manages to worm its way out, giving astronomers a key diagnostic of the protostar’s emergence.
The Journey from Protostar to Star
A protostar, however, is still not a star and the difference lies in the conditions at the core. A star like the Sun is powered by thermonuclear reactions. At the core of a mature star, such as the Sun, densities and temperatures are so high that hydrogen nuclei repeatedly slam together and fuse into helium, the next heaviest element on the periodic table. It takes four hydrogen nuclei (each of which is just one proton) to form a single helium nucleus (two protons and two neutrons). In the thermonuclear reactions that govern the transformation from hydrogen to helium, a small amount of the hydrogen’s mass is converted into energy (thanks to Einstein’s E = mc2). Stars, like the clouds they were born from, use thermal pressure to fight against their own crushing gravity. It’s the energy released in nuclear reactions that provides that pressure.
When a protostar begins to form at the center of a molecular cloudlet’s infalling maelstrom, it does not have central temperatures and densities high enough to trigger the Sun’s kind of nuclear reactions. Ironically, however, protostars can be much brighter than the Sun. Their luminosity is a temporary thing, borrowed from the energy of the initial cloudlet’s infall (those shock waves) and a slower contraction of the protostars’ mass. To truly become a mature star, protostars need to gain more mass from infall. They must also wait for contraction to drive the core density upward and increase central temperatures above the critical 10 million degrees kelvin where steady helium-producing thermonuclear reactions can begin.
If gravitational infall were all there was to star formation, astronomers would have enough to deal with. The birth of stars, however, involves another key player beyond just gravity and thermal pressure. Angular momentum, which you can think of as spin, not only changes the basic story of star formation—it’s also the reason why you and I are here standing on a planet to tell the tale.
The turbulence of molecular clouds means that every cloudlet that’s ready to gravitationally collapse is already spinning in one direction or another (think of all the spinning eddies when you vigorously stir a tub of water). Angular momentum is a measure of the cloudlet’s spin, and the fundamental laws of physics tell us that it is conserved. That means when a cloudlet changes size by collapsing, it can’t lose (or gain) any of its initial spin momentum. The conservation of angular momentum has important consequences for our star-formation story. Everyone who has ever watched Olympic figure skating has seen the principle in action: When a skater goes into a spin with outstretched arms and then pulls their arms inward, their rate of spin increases. That’s the conservation of angular momentum. If you are spinning and you get smaller, you have to also spin faster. The thing to notice, though, is how much faster the skater revolves after just changing their size by a single arm’s length. When a spinning cloudlet collapses to a protostar, it will shrink by a factor of 1 million or more. This is more than enough to reshape the architecture of the collapse.
When a spinning cloudlet collapses, all the gas does not fall straight onto the protostar. Instead, the axis of the spin defines an “equator” for the cloudlet (the equator would be perpendicular to the spin axis). Infalling rotating material coming from cloudlets’ equatorial regions can’t fall directly onto the star. Instead, it ends up moving around the spin axis so fast that it goes into orbit around the protostar rather than falling onto it. In this way, a disk of gas and dust forms around the protostar. This structure, called an accretion disk, will serve as a reservoir of material that slowly spirals through the disk and eventually makes it into the protostar. While originally a theoretical construct (demanded by the conservation of angular momentum), astronomers using the ALMA radio telescope array now have stunning high-resolution images of these disks in star-forming regions around the galaxy.

The appearance of those disks links directly to the appearance of astronomers like me who study them. Accretion disks are where planets form. As the dust and gas orbit the protostar a long series of interactions begin. Dust grains collide to form bigger dust grains, which then collide to form pebbles, which then form rocks and then boulders, and so on, all the way up to planets. Obviously, there is more to the planet-building process than this.
The story of planet formation is complicated, fascinating, and still getting worked out. But the important point for our story of star formation is that, while it begins with a light-year-spanning cloud of interstellar gas, it ends with planets. And on some of those planets, life will form, which is why the story of star formation really ends not just with planets but with you and me.
See the full article for Resources with links.
See the full article here.
Comments are invited and will be appreciated, especially if the reader finds any errors which I can correct.
five-ways-keep-your-child-safe-school-shootings
Please help promote STEM in your local schools.
JSTOR [Journal Storage] is a digital library of academic journals, books, and primary sources founded in 1994. Originally containing digitized back issues of academic journals, it now encompasses books and other primary sources as well as current issues of journals in the humanities and social sciences. It provides full-text searches of almost 2,000 journals. Most access is by subscription but some of the site is public domain, and open access content is available free of charge.
William G. Bowen, president of Princeton University from 1972 to 1988, founded JSTOR in 1994. JSTOR was originally conceived as a solution to one of the problems faced by libraries, especially research and university libraries, due to the increasing number of academic journals in existence. Most libraries found it prohibitively expensive in terms of cost and space to maintain a comprehensive collection of journals. By digitizing many journal titles, JSTOR allowed libraries to outsource the storage of journals with the confidence that they would remain available long-term. Online access and full-text searchability improved access dramatically.
Bowen initially considered using CD-ROMs for distribution. However, Ira Fuchs, Princeton University’s vice president for Computing and Information Technology, convinced Bowen that CD-ROM was becoming an increasingly outdated technology and that network distribution could eliminate redundancy and increase accessibility. (For example, all Princeton’s administrative and academic buildings were networked by 1989; the student dormitory network was completed in 1994; and campus networks like the one at Princeton were, in turn, linked to larger networks such as BITNET and the Internet.) JSTOR was initiated in 1995 at seven different library sites, and originally encompassed ten economics and history journals. JSTOR access improved based on feedback from its initial sites, and it became a fully searchable index accessible from any ordinary web browser. Special software was put in place to make pictures and graphs clear and readable.
With the success of this limited project, Bowen, Fuchs,and Kevin Guthrie, the then-president of JSTOR, wanted to expand the number of participating journals. They met with representatives of the Royal Society (UK) and an agreement was made to digitize the Philosophical Transactions of the Royal Society dating from its beginning in 1665. The work of adding these volumes to JSTOR was completed by December 2000. In 1999 JSTOR started a partnership with the Joint Information Systems Committee (UK) and created a mirror website at the University of Manchester (UK) to make the JSTOR database available to over 20 higher education institutions in England, Scotland, Wales and Northern Ireland.
The Andrew W. Mellon Foundation funded JSTOR initially. Until January 2009, JSTOR operated as an independent, self-sustaining nonprofit organization with offices in New York City and in Ann Arbor, Michigan. Then JSTOR merged with the nonprofit Ithaka Harbors, Inc.—a nonprofit organization founded in 2003 and “dedicated to helping the academic community take full advantage of rapidly advancing information and networking technologies”.
JSTOR content is provided by more than 900 publishers. The database contains more than 12 million journal articles, in more than 75 disciplines. Each object is uniquely identified by an integer value, starting at 1 which is used to create a stable URL.
In addition to the main site, the JSTOR labs group operates an open service that allows access to the contents of the archives for the purposes of corpus analysis at its Data for Research service. This site offers a search facility with graphical indication of the article coverage and loose integration into the main JSTOR site. Users may create focused sets of articles and then request a dataset containing word and n-gram frequencies and basic metadata. They are notified when the dataset is ready and may download it in either .XML or .CSV formats. The service does not offer full-text, although academics may request that from JSTOR, subject to a non-disclosure agreement.
JSTOR Plant Science is available in addition to the main site. JSTOR Plant Science provides access to content such as plant type specimens, taxonomic structures, scientific literature, and related materials and aimed at those researching, teaching, or studying botany, biology, ecology, environmental, and conservation studies. The materials on JSTOR Plant Science are contributed through the Global Plants Initiative (GPI) and are accessible only to JSTOR and GPI members. Two partner networks are contributing to this: the African Plants Initiative, which focuses on plants from Africa, and the Latin American Plants Initiative, which contributes plants from Latin America.
JSTOR launched its Books at JSTOR program in November 2012, adding 15,000 current and backlist books to its site. The books are linked with reviews and from citations in journal articles.
In September 2014, JSTOR launched JSTOR Daily, an online magazine meant to bring academic research to a broader audience. Posted articles are generally based on JSTOR entries, and some entries provide the backstory to current events.
Reveal Digital is a JSTOR-hosted collection of documents produced by or about underground, marginalized and dissenting 20th century communities. Reveal Digital’s open access content includes magazines, prison newspapers, AIDS art, student-movement documents, black civil rights materials, and a white supremacy archive.
JSTOR is licensed mainly to academic institutions, public libraries, research institutions, museums, and schools. More than 7,000 institutions in more than 150 countries have access. JSTOR has been running a pilot program of allowing subscribing institutions to provide access to their alumni, in addition to current students and staff. The Alumni Access Program officially launched in January 2013. Individual subscriptions also are available to certain journal titles through the journal publisher. Every year, JSTOR blocks 150 million attempts by non-subscribers to read articles.
Inquiries have been made about the possibility of making JSTOR open access. According to a Harvard Law professor, JSTOR had been asked “how much would it cost to make this available to the whole world, how much would we need to pay.”
The availability of most journals on JSTOR is controlled by a “moving wall”, which is an agreed-upon delay between the current volume of the journal and the latest volume available on JSTOR. This time period is specified by agreement between JSTOR and the publisher of the journal, which usually is three to five years. Publishers may request that the period of a “moving wall” be changed or request discontinuation of coverage. Formerly, publishers also could request that the “moving wall” be changed to a “fixed wall”—a specified date after which JSTOR would not add new volumes to its database. As of November 2010, “fixed wall” agreements were still in effect with three publishers of 29 journals made available online through sites controlled by the publishers.
In 2010, JSTOR started adding current issues of certain journals through its Current Scholarship Program.
Beginning September 6, 2011, JSTOR made public domain content available at no charge to the public. This “Early Journal Content” program constitutes about 6% of JSTOR’s total content, and includes over 500,000 documents from more than 200 journals that were published before 1923 in the United States, and before 1870 in other countries. JSTOR stated that it had been working on making this material free for some time. As of 2017, JSTOR does not have plans to extend it to other public domain content, stating that “We do not believe that just because something is in the public domain, it can always be provided for free”.
In January 2012, JSTOR started a pilot program, “Register & Read”, offering limited no-cost access (not open access) to archived articles for individuals who register for the service. At the conclusion of the pilot, in January 2013, JSTOR expanded “Register & Read” from an initial 76 publishers to include about 1,200 journals from over 700 publishers. Registered readers may read up to six articles online every calendar month, but may not print or download .PDFs.
In 2013, more than 8,000 institutions in more than 160 countries had access to JSTOR.
As of 2014, JSTOR is conducting a pilot program with Wikipedia, whereby established editors are given reading privileges through the Wikipedia Library, as with a university library.
In 2012, JSTOR users performed nearly 152 million searches, with more than 113 million article views and 73.5 million article downloads. JSTOR has been used as a resource for linguistics research to investigate trends in language use over time and also to analyze gender differences and inequities in scholarly publishing, revealing that in certain.
JSTOR metadata is available through CrossRef and the Unpaywall dump, which as of 2020 identifies nearly 3 million works hosted by JSTOR as toll access, as opposed to over 200,000 available in open access (mainly through third party open access repositories).