From Ethan Siegel
May 28, 2018
The black hole at the center of our Milky Way, simulated here, is the largest one seen from Earth’s perspective. The Event Horizon Telescope should, this year, come out with their first image of what this central black hole’s event horizon looks like. The white circle represents the Schwarzschild radius of the black hole. Ute Kraus, Physics education group Kraus, Universität Hildesheim; background: Axel Mellinger
Across multiple continents, including Antarctica, an array of radio telescopes observe the galactic center.
A view of the different telescopes contributing to the Event Horizon Telescope’s imaging capabilities from one of Earth’s hemispheres. The data taken from 2011 to 2017 should enable us to now construct an image of Sagittarius A*.
This network, the Event Horizon Telescope (EHT), is imaging, for the first time, a black hole’s event horizon.
The most-visualized black hole of all, as illustrated in the movie Interstellar, shows a predicted event horizon fairly accurately for a very specific class of rotating black holes. Interstellar / R. Hurt / Caltech
Of all the black holes visible from Earth, the largest is at the galactic center: 37 μas [Microarc-second].
This multiwavelength view of the Milky Way’s galactic center goes from the X-ray through the optical and into the infrared, showcasing Sagittarius A* and the intragalactic medium located some 25,000 light years away. Using radio data, the EHT will resolve the event horizon of the central black hole. X-ray: NASA/CXC/UMass/D. Wang et al.; Optical: NASA/ESA/STScI/D.Wang et al.; IR: NASA/JPL-Caltech/SSC/S.Stolov


With a theoretical resolution of 15 μas, the EHT should resolve it.
Despite the incredible news that they’ve detected the black hole’s structure at the galactic center, however, there’s still no direct image.
A plot of the coverage in space of the area around the galactic center’s black hole from the telescopes whose data has been brought together so far. Additional telescopes will further constrain the black hole’s size, shape and orientation. R.-S. Lu et al, ApJ 859, 1
They found evidence for an asymmetric source, about 3 Schwarzschild radii large: consistent with Einstein’s prediction of 2.5.
Two of the possible models that can successfully fit the Event Horizon Telescope data thus far. Both show an off-center, asymmetric event horizon that’s enlarged versus the Schwarzschild radius, consistent with the predictions of Einstein’s General Relativity. R.-S. Lu et al, ApJ 859, 1
But before the South Pole data, delivered five months ago, can be added, all error sources must be identified.
The South Pole Telescope, a 10 meter radio telescope located at the South Pole, will be the most important addition to the EHT as far as resolving the central black hole goes.
Earth’s atmospheric turbulence, instrumentation noise, and spurious signals require identification, obtainable through additional imaging.
A map of the 7 million second exposure of the Chandra Deep Field-South. This region shows hundreds of supermassive black holes, each one in a galaxy far beyond our own. There should be hundreds of thousands of times as many stellar-mass black holes; we’re just waiting for the capability of detecting them. NASA/CXC/B. Luo et al., 2017, ApJS, 228, 2
Although the data has been combined, novel algorithms must be developed to process them into an image.
Five different simulations in general relativity, using a magnetohydrodynamic model of the black hole’s accretion disk, and how the radio signal will look as a result. Note the clear signature of the event horizon in all the expected results. GRMHD simulations of visibility amplitude variability for Event Horizon Telescope images of Sgr A*, L. Medeiros et al., arXiv:1601.06799
Only two black holes, Sagittarius A* and Messier 87, could have event horizon “silhouettes” imaged.
The second-largest black hole as seen from Earth, the one at the center of the galaxy Messier 87, is shown in three views here. Despite its mass of 6.6 billion Suns, it is over 2000 times farther away than Sagittarius A*. It may not be resolvable by the EHT.Top, optical, Hubble Space Telescope / NASA / Wikisky; lower left, radio, NRAO / Very Large Array (VLA); lower right, X-ray, NASA / Chandra X-ray telescope

New data will be taken annually, improving the future, overall pictures through subsequent analysis.
Arizona Radio Observatory/Submillimeter-wave Astronomy (ARO/SMT)
Combined Array for Research in Millimeter-wave Astronomy (CARMA)
Atacama Submillimeter Telescope Experiment (ASTE)
Caltech Submillimeter Observatory (CSO)
Institut de Radioastronomie Millimetrique (IRAM) 30m
James Clerk Maxwell Telescope interior, Mauna Kea, Hawaii, USA
Large Millimeter Telescope Alfonso Serrano
Submillimeter Array Hawaii SAO
ESO/NRAO/NAOJ ALMA Array, Chile
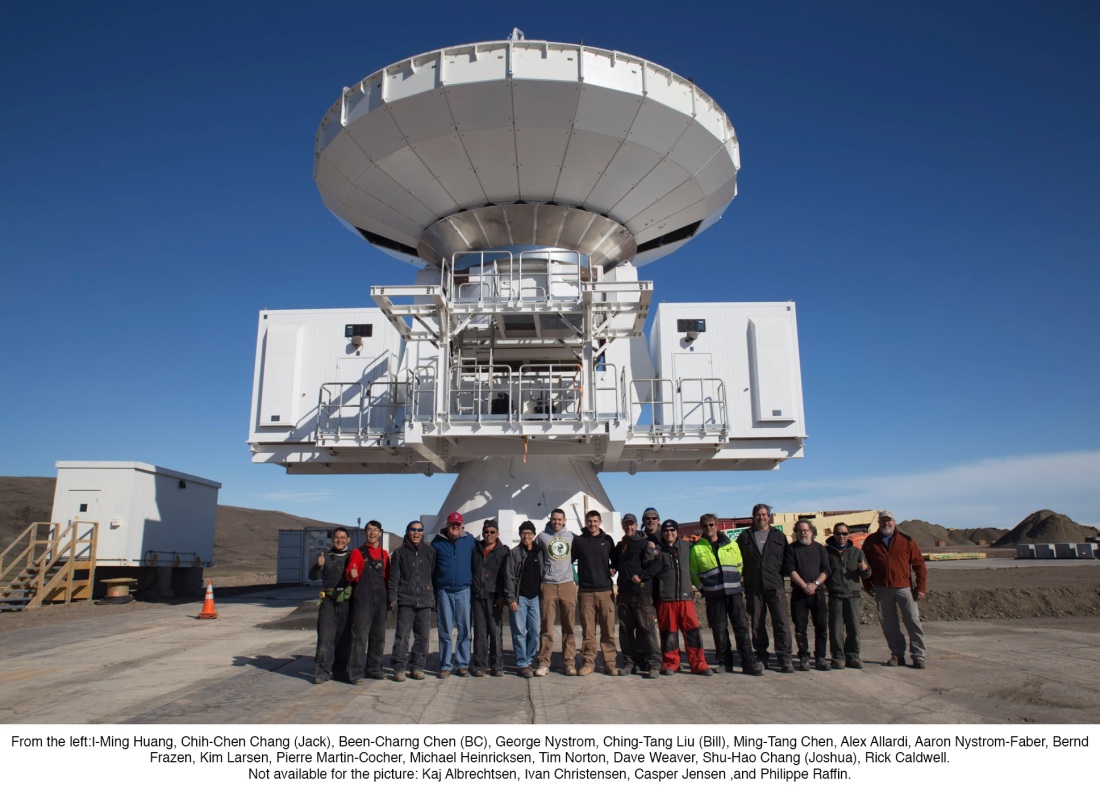
Greenland Telescope
Future Array/Telescopes
Plateau de Bure interferometer
Over the coming months, preliminary images will show the:
size,
shape,
changes,
and surrounding environment,
of our first directly-observed black holes.
High-Angular-Resolution and High-Sensitivity Science Enabled by Beamformed ALMA, V. Fish et al., arXiv:1309.3519
Some of the possible profile signals of the black hole’s event horizon as simulations of the Event Horizon Telescope indicate.
See the full article here .
five-ways-keep-your-child-safe-school-shootings
Please help promote STEM in your local schools.
Stem Education Coalition
“Starts With A Bang! is a blog/video blog about cosmology, physics, astronomy, and anything else I find interesting enough to write about. I am a firm believer that the highest good in life is learning, and the greatest evil is willful ignorance. The goal of everything on this site is to help inform you about our world, how we came to be here, and to understand how it all works. As I write these pages for you, I hope to not only explain to you what we know, think, and believe, but how we know it, and why we draw the conclusions we do. It is my hope that you find this interesting, informative, and accessible,” says Ethan